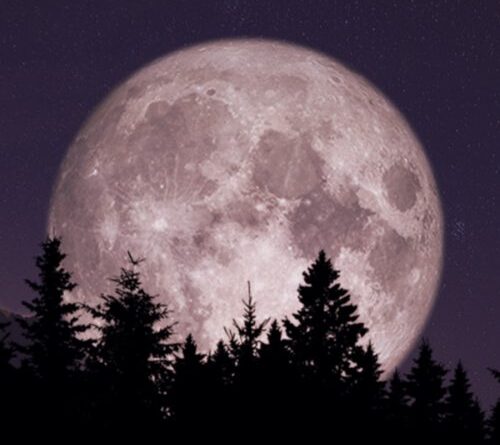
Getting oxygen from regolith takes 24 kWh per kg, and we ‘d require tonnes.
Without modifications for relativity, clocks here and on the Moon would quickly diverge.
Credit: NASA
If mankind is ever to expand into the Solar System, we’re going to require to discover a method to put fuel into rockets someplace besides the comfortable boundaries of a launchpad in the world. One alternative for that remains in low-Earth orbit, which has the benefit of liing really near to stated launch pads. It has the significant downside of needing a lot of energy to leave Earth’s gravity– it takes a lot of fuel to put significantly less fuel into orbit.
One option is to produce fuel on the Moon. We understand there is hydrogen and oxygen present, and the Moon’s gravity is far much easier to conquer, indicating more of what we produce there can be utilized to send out things much deeper into the Solar System. There is a tradeoff: Any fuel-production facilities will likely require to be developed on Earth and sent out to the Moon.
Just how much facilities is that going to include? A research study launched today by PNAS assesses the energy expenses of producing oxygen on the Moon and discovers that they’re considerable: about 24 kWh per kg. This does not sound bad up until you begin thinking about the number of kgs we’re going to ultimately require.
Free the oxygen!
The mathematics that makes refueling from the Moon appealing is quite easy. “As a rule of thumb,” compose the authors of the brand-new research study on the subject, “rockets launched from Earth destined for [Earth-Moon Lagrange Point 1] must burn ~25 kg of propellant to transport one kg of payload, whereas rockets launched from the Moon to [Earth-Moon Lagrange Point 1] would burn only ~four kg of propellant to transport one kg of payload.” Leaving from the Earth-Moon Lagrange Point for places deeper into the Solar System likewise needs less energy than leaving low-Earth orbit, indicating the fuel we arrive is eventually better, a minimum of from an expedition viewpoint.
Of course, you require to make the fuel there in the very first location. The apparent option for that is water, which can be divided to produce hydrogen and oxygen. We understand there is water on the Moon, however we do not yet understand just how much, and whether it’s focused into big deposits. Considered that unpredictability, individuals have actually likewise taken a look at other products that we understand exist in abundance on the Moon’s surface area.
And there’s most likely absolutely nothing more plentiful on that surface area than regolith, the dust left over from continuous small effects that have, gradually, wore down lunar rocks. The regolith is made up of a range of minerals, much of which consist of oxygen, normally the much heavier part of rocket fuel. And a range of individuals have actually found out the chemistry associated with separating oxygen from these minerals on the scale required for rocket fuel production.
Understanding the chemistry is various from understanding what sort of facilities is required to get that chemistry done at a significant scale. To get a sense of this, the scientists chose to concentrate on separating oxygen from a mineral called ilmenite, or FeTiO3It’s not the most convenient method to get oxygen– iron oxides triumph there– however it’s well comprehended. Somebody really patented oxygen production from ilmenite back in the 1970s, and 2 hardware models have actually been established, among which might be sent out to the Moon on a future NASA objective.
The scientists propose a system that would collect regolith, partially cleanse the ilmenite, then integrate it with hydrogen at heats, which would remove the oxygen out as water, leaving cleansed iron and titanium (both of which might work to have). The resulting water would then be divided to feed the hydrogen back into the system, while the oxygen can be dispatched for usage in rockets.
(This would not resolve the problem of what that oxygen will eventually oxidize to power a rocket. Oxygen is generally the much heavier part of rocket fuel mixes– normally about 80 percent of the mass– and so, is the larger obstacle to get to a fuel depot.)
Certainly, this procedure will need a great deal of facilities, like harvesters, separators, high-temperature response chambers, and more. The scientists focus on a single aspect: how much power will it draw down?
More power!
To get their numbers, the scientists made a couple of streamlining presumptions. These consist of presuming that it’s possible to cleanse ilmenite from raw regolith which it will exist in particles little enough that about half the product present will take part in chain reactions. They neglected both the prospective to get back at more oxygen from the iron and titanium oxides present, along with the capacity for contamination from bothersome products like hydrogen sulfide or hydrochloric acid.
The group discovered that practically all of the energy is taken in at 3 actions in the procedure: the high-temperature hydrogen response that produces water (55 percent), splitting the water later (38 percent), and transforming the resulting oxygen to its liquid type (5 percent). The normal overall use, depending upon aspects like the concentration of ilmenite in the regolith, exercised to be about 24 kW-hr for each kg of liquid oxygen.
Undoubtedly, the numbers are delicate to how effectively you can do things like heat the response mix. (It may be possible to do this heating with focused solar, preventing making use of electrical power for this completely, however the authors didn’t examine that.) It was likewise delicate to less apparent performances. A much better separation of the ilmenite from the rest of the regolith indicates you’re utilizing less energy to heat pollutants. While the energetic expense of that separation is little, it pays off to do it efficiently.
Based upon orbital observations, the scientists draw up the locations where ilmenite exists at high adequate concentrations for this technique to make good sense. These consist of a few of the mares on the near side of the Moon, so they’re simple to get to.
A map of the lunar surface area, with locations with high ilmenite concentrations displayed in blue.
Credit: Leger, et. al.
A map of the lunar surface area, with locations with high ilmenite concentrations displayed in blue.
Credit: Leger, et. al.
By itself, 24 kWh does not appear like a great deal of power. The issue is that we will require a great deal of kgs. The scientists approximate that getting an empty SpaceX Starship from the lunar surface area to the Earth-Moon Lagrange Point takes 80 tonnes of liquid oxygen. And a totally sustained starship can hold over 500 tonnes of liquid oxygen.
We can compare that to something like the solar selection on the International Space Station, which has a capability of about 100 kW. That implies it might power the production of about 4 kgs of oxygen an hour. At that rate, it’ll take a bit over 10 days to produce a tonne, and a bit more than 2 years to get enough oxygen to get an empty Starship to the Lagrange Point– presuming 24-7 production. Being on the near side, they will just produce for half the time, offered the lunar day.
Undoubtedly, we can develop bigger varieties than that, however it increases the quantity of product that requires to be sent out to the Moon from Earth. It might possibly make more sense to utilize nuclear power. While that would likely include more facilities than solar varieties, it would enable the centers to run around the clock, hence getting more production from whatever else we’ve delivered from Earth.
This paper isn’t implied to be the last word on the possibilities for lunar-based refueling; it’s merely an early effort to put tough numbers on what eventually may be the very best method to explore our Solar System. Still, it offers some viewpoint on simply just how much effort we’ll require to make before that sort of expedition ends up being possible.
PNAS2025. DOI: 10.1073/ pnas.2306146122 (About DOIs).
John is Ars Technica’s science editor. He has a Bachelor of Arts in Biochemistry from Columbia University, and a Ph.D. in Molecular and Cell Biology from the University of California, Berkeley. When physically separated from his keyboard, he tends to look for a bike, or a picturesque place for communicating his treking boots.
177 Comments
Find out more
As an Amazon Associate I earn from qualifying purchases.