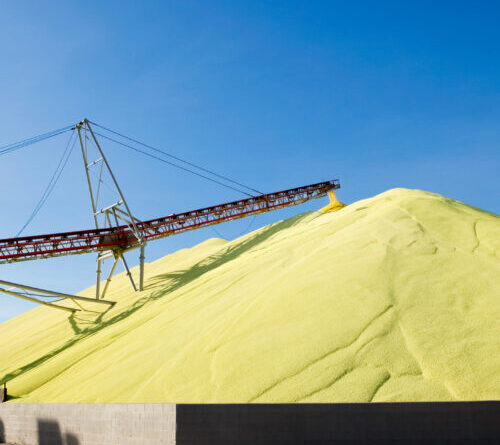
Sulfur can save a lot more lithium however is problematically reactive in batteries.
If you weren’t conscious, sulfur is quite plentiful.
Credit: P_Wei
Lithium might be the crucial element in many contemporary batteries, however it does not comprise the bulk of the product utilized in them. Rather, much of the product remains in the electrodes, where the lithium gets saved when the battery isn’t charging or releasing. One method to make lighter and more compact lithium-ion batteries is to discover electrode products that can keep more lithium. That’s one of the factors that current generations of batteries are beginning to include silicon into the electrode products.
There are products that can keep a lot more lithium than silicon; a significant example is sulfur. Sulfur has a propensity to respond with itself, producing ions that can drift off into the electrolyte. Plus, like any electrode product, it tends to broaden in percentage to the quantity of lithium that gets saved, which can produce physical stress on the battery’s structure. While it has actually been simple to make lithium-sulfur batteries, their efficiency has actually tended to deteriorate quickly.
This week, scientists explained a lithium-sulfur battery that still has over 80 percent of its initial capability after 25,000 charge/discharge cycles. All it took was a strong electrolyte that was more reactive than the sulfur itself.
When lithium fulfills sulfur …
Sulfur is an appealing battery product. It’s plentiful and inexpensive, and sulfur atoms are reasonably light-weight compared to a number of the other products utilized in battery electrodes. Sodium-sulfur batteries, which count on 2 really inexpensive basic materials, have actually currently been established, although they just operate at temperature levels high enough to melt both of these parts. Lithium-sulfur batteries, by contrast, might run basically the very same method that present lithium-ion batteries do.
With a couple of significant exceptions, that is. One is that the essential sulfur utilized as an electrode is a really bad conductor of electrical power, so it needs to be distributed within a mesh of conductive product. (You can contrast that with graphite, which both shops lithium and performs electrical energy reasonably well, thanks to being made up of many sheets of graphene.) Lithium is kept there as Li2S, which inhabits considerably more area than the essential sulfur it’s changing.
Both of these concerns, nevertheless, can be fixed with mindful engineering of the battery’s structure. A more serious issue originates from the residential or commercial properties of the lithium-sulfur responses that take place at the electrode. Essential sulfur exists as an eight-atom ring, and the responses with lithium are sluggish enough that semi-stable intermediates with smaller sized chains of sulfur wind up forming. These tend to be soluble in the majority of electrolytes, permitting them to take a trip to the opposite electrode and take part in chemical responses there.
This procedure basically releases the battery without enabling the electrons to be used. And it slowly leaves the electrode’s sulfur not available for taking part in future charge/discharge cycles. The net outcome is that early generations of the innovation would release themselves while sitting unused and would just endure a couple of hundred cycles before efficiency decomposed drastically.
There has actually been development on all these fronts, and some lithium-sulfur batteries with efficiency comparable to lithium-ion have actually been shown. Late in 2015, a business revealed that it had actually lined up the cash required to develop the very first massive lithium-sulfur battery factory. Still, deal with enhancements has actually continued, and the brand-new work appears to recommend methods to improve efficiency well beyond lithium-ion.
The requirement for speed
The paper explaining the brand-new advancements, done by a partnership in between Chinese and German scientists, concentrates on one element of the difficulties positioned by lithium-sulfur batteries: the reasonably sluggish chain reaction in between lithium ions and essential sulfur. It provides that element as an obstruction to quickly charging, something that will be a problem for automobile applications. At the very same time, discovering a method to restrict the development of non-active intermediate items throughout this response goes to the root of the fairly brief functional life period of lithium-sulfur batteries.
As it ends up, the scientists discovered 2.
Among the issues with the lithium-sulfur response intermediates is that they liquify in a lot of electrolytes. That’s not an issue if the electrolyte isn’t a liquid. Strong electrolytes are products that have a permeable structure at the atomic level, with the environment inside the pores agreeing with for ions. This permits ions to diffuse through the strong. If there’s a method to trap ions on one side of the electrolyte, such as a chain reaction that traps or de-ionizes them, then it can allow one-way travel.
Seriously, pores that prefer the transit of lithium ions, which are rather compact, aren’t most likely to permit the transit of the big ionized chains of sulfur. A strong electrolyte needs to assist cut down on the issues dealt with by lithium-sulfur batteries. It will not always assist with quick charging.
The scientists started by evaluating a glass formed from a mix of boron, sulfur, and lithium (B2S3 and Li2S). This glass had dreadful conductivity, so they began exploring with associated glasses and settled on a mix that replaced in some phosphorus and iodine.
The iodine ended up being an important element. While the exchange of electrons with sulfur is reasonably sluggish, iodine goes through electron exchange (technically called a redox response) incredibly rapidly. It can act as an intermediate in the transfer of electrons to sulfur, speeding up the responses that happen at the electrode. In addition, iodine has reasonably low melting and boiling points, and the scientists recommend there’s some proof that it moves within the electrolyte, permitting it to serve as an electron shuttle bus.
Successes and cautions
The outcome is a far remarkable electrolyte– and one that allows quickly charging. It’s normal that quick charging cuts into the overall capability that can be saved in a battery. When charged at an extremely quick rate (50C, indicating a complete charge in simply over a minute), a battery based on this system still had half the capability of a battery charged 25 times more gradually (2C, or a half-hour to complete charge).
The striking thing was how resilient the resulting battery was. Even at an intermediate charging rate (5C), it still had more than 80 percent of its preliminary capability after over 25,000 charge/discharge cycles. By contrast, lithium-ion batteries tend to strike that level of decay after about 1,000 cycles. If that sort of efficiency is possible in a mass-produced battery, it’s just a small exaggeration to state it can significantly modify our relationships with numerous battery-powered gadgets.
What’s not clear, nevertheless, is whether this makes the most of among the initial pledges of lithium-sulfur batteries: more charge in a provided weight and volume. The scientists define the battery being utilized for screening; one electrode is an indium/lithium metal foil, and the other is a mix of carbon, sulfur, and the glass electrolyte. A layer of the electrolyte sits in between them. When providing numbers for the storage capability per weight, just the weight of the sulfur is discussed.
Still, even if weight problems would prevent this from being packed into a vehicle or mobile phone, there are lots of storage applications that would gain from something that does not break even with 65 years of day-to-day biking.
Nature, 2025. DOI: 10.1038/ s41586-024-08298-9 (About DOIs).
John is Ars Technica’s science editor. He has a Bachelor of Arts in Biochemistry from Columbia University, and a Ph.D. in Molecular and Cell Biology from the University of California, Berkeley. When physically separated from his keyboard, he tends to look for a bike, or a beautiful area for communicating his treking boots.
99 Comments
Learn more
As an Amazon Associate I earn from qualifying purchases.